Brain sleep stages control orchestrates a complex relationship between neurological processes and physical well-being, shaping our daily functioning and long-term health. Sleep has emerged as a vital biological process throughout evolution, with different stages serving distinct restorative functions.
Sleep comprises distinct phases that progress from wakefulness through light and deep sleep, ultimately reaching rapid eye movement (REM) sleep. These stages work harmoniously, creating approximately 90-minute cycles that repeat throughout the night, each contributing uniquely to physical restoration and cognitive function.
Research reveals that early sleep cycles feature predominantly non-REM (NREM) sleep, whilst REM sleep dominates the latter portion of our slumber. This careful orchestration reflects the brain’s sophisticated control over sleep architecture, ensuring optimal physiological benefits from each sleep stage.
The brain demonstrates remarkable precision in regulating these sleep stages, exhibiting distinct electrical patterns and neural activities during different phases. These patterns range from the synchronised slow waves of deep sleep to the wake-like activation seen during REM sleep, each serving specific biological purposes.
Through advanced neuroscience research, we now understand that brain sleep stages control involves complex interactions between various brain regions, neurotransmitters, and hormones. This elaborate system maintains our physical well-being through mechanisms that influence everything from muscle recovery to immune function.
From electrical wave patterns shaping sleep quality to protective mechanisms safeguarding our rest, a fascinating journey through sleep’s complex architecture awaits.
Brain Sleep Stages Control Functional Architecture
The functional architecture of sleep reveals a complex system where NREM (Non-Rapid Eye Movement) and REM (Rapid Eye Movement) sleep alternate in approximately 90-minute cycles throughout the night.
During these cycles, brain sleep stages control mechanisms orchestrate transitions between three distinct NREM stages before entering REM sleep.
Early night sleep cycles demonstrate a fascinating predominance of NREM sleep, particularly its deepest phase (N3 or slow-wave sleep). As the night progresses, brain sleep stages control shifts, with REM sleep becoming increasingly prevalent.
This strategic timing ensures the optimal processing of different physiological needs. NREM Sleep Stages:
N1 (Light Sleep)
- Duration: 1-7 minutes
- Characteristics: Rhythmical alpha waves at 8-13 cycles per second
- Function: Transition between wakefulness and deeper sleep
N2 (Intermediate Sleep)
- Initial duration: 10-25 minutes
- Comprises 50% of total sleep time
- Features: Sleep spindles and K-complexes (brief bursts of brain activity)
- Primary role: Memory consolidation
N3 (Deep Sleep)
- Duration: 20-40 minutes
- Characteristics: High-voltage, slow-wave patterns
- Critical for: Physical restoration and growth hormone release
REM sleep presents a unique paradox, accounting for 20-25% of total sleep time. While the brain demonstrates intense activity patterns similar to wakefulness, the body experiences complete voluntary muscle paralysis (muscle atonia). This paralysis is a protective mechanism, preventing physical acting out of dreams.
The brain’s control over these sleep stages involves precise coordination between neural networks. The wake-promoting network, comprising several specialised neurons (cholinergic, noradrenergic, serotonergic), works in concert with sleep-promoting networks in the anterior hypothalamus (a region regulating sleep) to enable seamless transitions between sleep states.
The figure below illustrates this precise orchestration of sleep stages throughout a typical night. Sleep architecture follows a predictable pattern, with the brain cycling through different stages approximately every 90 minutes. Early cycles demonstrate a predominance of N3 (deep sleep), highlighted by extended periods in this stage.
As the night progresses, REM sleep periods (shown in light blue shading) become more frequent and prolonged, while N3 periods decrease. This systematic progression reflects the brain’s sophisticated control mechanisms, ensuring optimal restoration and cognitive processing at different points throughout our sleep period.
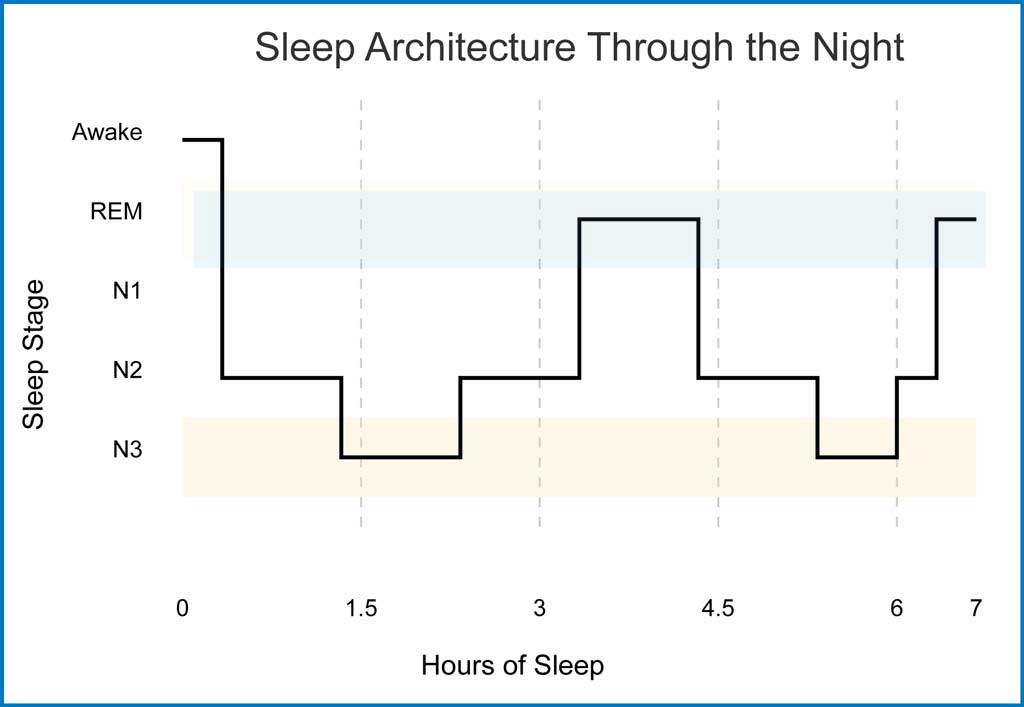
Electrical Patterns Shape Sleep Quality
The brain’s electrical activity undergoes remarkable transformations during different sleep phases, creating distinct patterns that define sleep quality. These patterns serve as windows into understanding how effectively our brain processes information and maintains physical well-being.
During wakefulness, brain activity displays fast, chaotic patterns. However, as sleep deepens, these patterns undergo significant changes:
Sleep Spindles (protective brain waves)
- Frequency: 12-14 Hz bursts
- Duration: 0.5-2 seconds
- Primary function: Shield brain from external disturbances
- Impact: Stronger spindles correlate with better sleep maintenance
Slow Wave Activity (SWA)
- Origin: Middle-frontal brain regions
- Movement: Front-to-back wave propagation
- Characteristics: Synchronised firing of thousands of neurons
- Role: Critical for memory processing and storage
The thalamus (a brain structure that acts as a relay station) plays a crucial role in modulating these electrical patterns. During sleep, it functions as a sensory gate, actively blocking external signals from reaching the cortex (the brain’s outer layer). This gating mechanism enables the brain to:
- Maintain sleep continuity
- Process internal information
- Facilitate memory consolidation
- Support physical restoration
Brain wave patterns demonstrate fascinating changes across different brain sleep stages control:
- Light Sleep: Alpha waves transition to theta waves
- Deeper Sleep: Delta waves become prominent
- REM Sleep: Mixed-frequency waves similar to wakefulness
Research indicates that these electrical patterns directly influence physical well-being through their effects on hormone release, immune function, and cellular repair. The synchronised slow-wave activity during deep sleep mainly correlates with growth hormone secretion and tissue regeneration.
Brain Sleep Stages Control Protective Mechanisms
Brain sleep stages control incorporates sophisticated protective mechanisms safeguarding our physical well-being during rest. One remarkable mechanism involves complete muscle paralysis during REM sleep, where the brain stem sends inhibitory signals down the spinal cord to prevent dream enactment.
Sleep spindles act as neurological guardians during sleep:
- Function: Shield brain from external disturbances
- Location: Generated in the thalamus (a brain region that relays sensory information)
- Timing: Occurs during NREM (Non-Rapid Eye Movement) sleep
- Impact: Higher spindle frequency correlates with better sleep preservation
The brain sleep stages control system employs multiple protective strategies during different sleep phases:
NREM Sleep Protection
- Reduced sensory processing
- Decreased motor reflexes
- Lowered brain temperature
- Regular breathing patterns
- Stabilised blood pressure
REM Sleep Protection
- Complete muscle atonia (paralysis)
- Increased brain activity
- Variable autonomic function
- Rapid eye movements
- Heightened arousal threshold
The thalamus is a crucial protective gateway, actively filtering incoming sensory information during sleep. This selective barrier ensures that only significant stimuli can prompt awakening while routine environmental noise remains blocked. Research shows this filtering mechanism becomes increasingly effective as sleep deepens.
Sleep Pressure and Circadian Rhythm Partnership
The elaborate partnership between sleep pressure and circadian rhythms represents a key component of brain sleep stages control. This dual control system involves homeostatic sleep pressure (which builds during wakefulness) and circadian timing (our internal 24-hour clock).
The hypothalamus houses the suprachiasmatic nucleus (SCN), often called the master biological clock, which coordinates various physiological processes. Core Clock Functions:
- Hormone regulation
- Temperature control
- Sleep-wake patterns
- Metabolic processes
- Cognitive performance
Environmental cues, exceptionally light exposure, significantly influence this system:
- Light information travels via melanopsin cells (specialised retinal cells)
- Signals reach multiple brain regions
- Impacts alertness and mood
- Regulates seizure susceptibility
- Influences migraine sensitivity
Temperature regulation plays a vital role in sleep initiation:
- Core body temperature must decrease by 2-3°F (1°C)
- Thermosensitive cells detect this change
- Signals trigger evening melatonin surge
- Both declining light and temperature coordinate sleep timing
Complex brain sleep stages control mechanisms coordinate temperature regulation through three key areas:
- Hands: Dense networks of blood vessels near the skin surface
- Feet: Specialised heat-loss vessels
- Head: Efficient cooling mechanisms through arteriovenous anastomoses
Sleep has overwhelmingly shaped our species’ journey through time. From the evolution of NREM sleep to the later development of REM sleep in birds and mammals, these stages have become increasingly sophisticated.
This biological symphony continues to orchestrate our daily restoration, weaving together threads of consciousness and unconsciousness in an endless dance of renewal and rejuvenation.
Sources
- Achermann P, Borbély AA (1997). Low-frequency (1 Hz) oscillations in the human sleep electroencephalogram. Neuroscience, 81, 213–222.
- Berry RB, Brooks R, Gamaldo C, Harding SM, Lloyd RM, Quan SF, Troester MT, Vaughn BV. AASM Scoring Manual Updates for 2017 (Version 2.4). J Clin Sleep Med. 2017 May 15;13(5):665-666.
- Bassetti CL, Hermann DM (2010). Sleep and stroke. Handbook of Clinical Neurology: Sleep Disorders. New York: Elsevier.
- Born J, Rasch B, Gais S (2006). Sleep to remember. Neuroscientist, 12, 410–424.
- Braun AR, Balkin TJ, Wesenten NJ, Carson RE, Varga M, Baldwin P, Selbie S, Belenky G, Herscovitch P. Regional cerebral blood flow throughout the sleep-wake cycle. An H2(15)O PET study. Brain. 1997 Jul;120 ( Pt 7):1173-97.
- Berson D, et al. (2002). Phototransduction by retinal ganglion cells that set the circadian clock. Science, 295, 1070-4.
- Cantero JL, Atienza M, Stickgold R (2003). Sleep-dependent theta oscillations in the human hippocampus and neocortex. J Neurosci, 23, 10897–10903.
- Daan S, Beersma DG, Borbély AA (1984). Timing of human sleep: recovery process gated by circadian pacemaker. Am J Physiol, 246, R161-83.
- Dement W, & Kleitman N (1957). Cyclic variations in EEG during sleep and their relations to eye movements, body motility, and dreaming. Electroencephalography and Clinical Neurophysiology, 9, 673–690.
- Fuller PM, Gooley JJ, Saper CB (2006). Neurobiology of the sleep-wake cycle: sleep architecture, circadian regulation, and regulatory feedback. J Biol Rhythms, 21, 482-93.
- Howard S, Gaba DM, Rosekind MR (2002). The risk and implications of excessive daytime sleepiness in resident physicians. Acad Med, 77, 1019–25.
- Institute of Medicine (2006). Sleep disorders and sleep deprivation: an unmet public health problem. Washington, DC: The National Academies Press.
- Kryger M, Roth T, Dement WC (2017). Principles and Practice of Sleep Medicine. 6th ed. Philadelphia, PA: Elsevier.
- Landry GJ, Best JR, Liu-Ambrose T (2015). Measuring sleep quality in older adults: a comparison using subjective and objective methods. Front Aging Neurosci, 7, 166.
- McCarley RW (2007). Neurobiology of REM and NREM Sleep. Sleep Med, 8, 302–330.
- McNicholas WT, Krieger J, Levy P (2002). Public health and medicolegal implications of sleep apnoea. Eur Respir J, 20, 1594–609.
- Peever J, Fuller PM (2017). The Biology of REM Sleep. Curr Biol, 27, R1237–R1248.
- Richter C, Woods IG, Schier AF (2014). Neuropeptidergic control of sleep and wakefulness. Annu Rev Neurosci, 37, 503-31.
- Siegel JM (2005). Clues to the Functions of Mammalian Sleep. Nature, 437, 1264–1271.
- Siegel JM, Moore R, Thannickal T (2001). A brief history of hypocretin/orexin and narcolepsy. Neuropsychopharmacology, 25(5), S14-20.
- Stickgold R, & Walker MP (2005). Memory consolidation and reconsolidation: What is the role of sleep? Trends in Neuroscience, 28, 408-415.
- Tranah GJ, Blackwell T, Stone KL (2011). Circadian activity rhythms and risk of incident dementia and mild cognitive impairment in older women. Ann Neurol, 70(5), 722–32.